Spinal Cord Stimulation Sees Paralyzed Up And Walking, But Scientists Still Frustrated With Current Technology
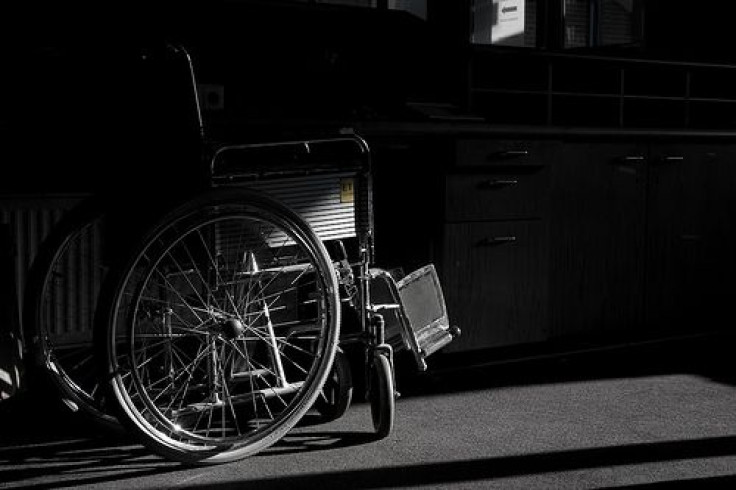
While some victims of paralysis have come to embrace the wheelchair they now occupy, some still view the vehicle as a daily reminder that their motor functions aren’t what they used to be. But to add that “and they never will be either” may be overly presumptuous, as a bulk of scientific research now points to spinal cord stimulation (SCS) as the new frontier for getting people back on their feet — and more importantly, staying there.
SCS as a means to rehabilitate the paralyzed has been in use since the 1970s, when scientists pumped electricity by the truckload into patients’ spinal cords just to get them to take a single step. Since then, the process has become more refined. In 2011, a breakthrough was made by University of Louisville neuroscientist Dr. Susan Harkema when she restored a paralyzed man’s bladder, bowel, and sexual function through lower SCS on the first day of treatment. Now, Harkema has a handful of subjects on whom she tests the new procedure with promising results.
Modern incarnations of SCS rely on the body’s own processes rather than sheer force from the outside. Spinal cord neurons don’t receive signals solely from the brain: when a muscle moves, or a person centers her balance, the resulting feedback gets sent to the neurons. These processes help regulate movement in the same way scientists once thought could only be accomplished through high-voltage stimulation.
“It’s like putting a hearing aid on the spinal cord,” neurobiologist V. Reggie Edgerton, Harkema’s former mentor and current collaborator in the Louisville experiments, told IEEE Spectrum. “We’ve changed the physiological properties of the neural network so that now it can ‘hear’ the sensory information much better and can learn what to do with it.”
Admittedly, the first patient, Rob Summers, may have been an anomaly. His improvement outpaced even the most industrious lab rats. Still, it merited attention from the Food and Drug Administration (FDA), who granted Harkema four more patients to continue her studies. One of those patients was an outdoorsy Wyoming native named Dustin Shillcox.
Shillcox suffered, among other traumatic injuries, a broken back. His recovery was slow, and despite infections eventually dissipating and bones healing, he left the hospital paralyzed from the chest down.
Shillcox hasn’t enjoyed a rehab time as immediate as Summers. In fact, progress has been somewhat stagnant. But it’s not a matter of personal effort or scientific unknowns holding him back. Frankly, Harkema says, it’s the equipment.
Exploring The Possibilities
Paralysis is the result of blocked communication between the brain and spinal cord, more specifically in the bundle of neurons at the base of the spinal cord that coordinates with muscular movement. This means that if scientists can send a signal directly from that neural bundle, they can avoid worrying about the brain altogether. Unfortunately, the technology that taps into these neurons isn’t as sophisticated as it needs to be.
Harkema’s lab uses a Medtronic — a device used for pain management that’s approved by the FDA — that uses 16 electrode sensors surgically implanted in the subject’s epidural space near the spinal cord. Operating the device is simple, but operating it effectively is exhausting. Seventy-five minutes into trial-and-error combinations of electrodes — Harkema’s only option for deducing which connections produce the action of stepping — she finally removes the bungee cord that’s been supporting Shillcox’s weight. Now he stands. The cord had been bearing too great a load. She decided to try again the next day, without the cord.
Using the device to supply electricity to Shillcox’s spinal cord is tiresome mostly because Harkema must reset the machine to zero volts after each unsuccessful attempt. The electric field needs the safety for precautionary measures, but she argues it slows neural momentum. “You can get really close, and you think the person is almost standing independently, and if you could just shift the field a little you would have it,” she said. “But you can’t. You have to go to zero. And then everything starts over.”
The team has been dabbling in other alternatives. They’ve collaborated with California Institute of Technology engineer Joel Burdick to design an algorithm that removes some of the guesswork in choosing which electrodes to activate. They’ve also enlisted the help of electrical engineer John Naber, of the University of Louisville, who is working on a way to give Harkema independent control of the Medtronic. It’ll allow her to operate the machine without having to keep resetting it between attempts. Here, the challenge is FDA approval.
“It’s not like a commercial integrated circuit or product, because of the FDA requirements for human implants,” Naber told IEEE Spectrum.
Another obstacle is replacing the Medtronic altogether with something better suited to their goals. The device is meant to treat pain, so its current is emitted more broadly than is desirable. Again, the fixes are in the works.
Harkema’s other two subjects have completed their initial trials, standing up successfully and affirming for Harkema that her method is moving forward, if sluggishly. She and her team haven’t announced yet whether the same functions have returned in these subjects that returned in Summers. For Shillcox, at least, the prospect of getting out of his chair — which, he says, has been a great source of consternation — is one he’s very much looking forward to.
“I don’t want to be too optimistic, and I’m trying to be prepared for no results at all,” he said. “I hope that whatever they find from this research will at least benefit other people.”