Reversing Memory Loss in Snails May Lead to Same in Humans
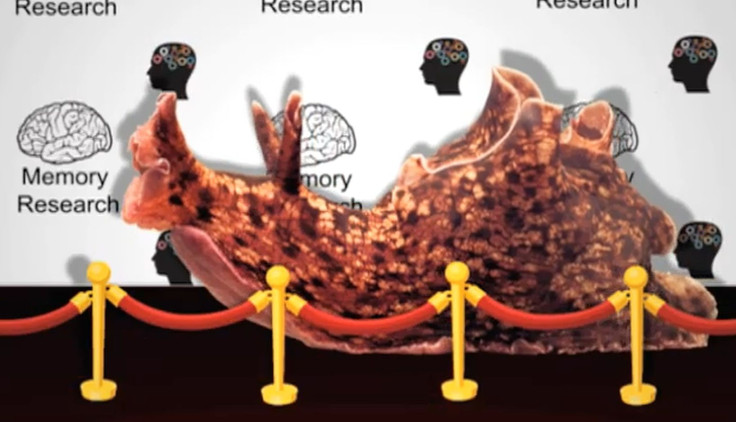
Neuroscientists have reversed memory loss in sea snail nerve cells— a development that may eventually lead to treatments for age-related memory decline, intellectual disabilities, and degenerative brain diseases like Alzheimer's in humans.
Researchers at the University of Texas Health Science Center at Houston (UTHealth) developed a mathematical model that predicted when molecular processes in the sea snail brain were most sensitive to learning. Using that model, they were able to schedule ideal times to retrain nerve cells after their connections were weakened to induce memory loss.
The study, published in the April 17 issue of The Journal of Neuroscience, is a proof-of-concept that shows how a similar process could work in live animals.
"Although much works remains to be done, we have demonstrated the feasibility of our new strategy to help overcome memory deficits," said Dr. Jack Byrne, the study's senior author, in a news release.
The sea snail species used in the research, Aplysia californica, has a simple nervous system with a relatively small number of brain cells. It makes an excellent model for brain research because its neurons share similar properties to those of humans and are enormous— at up to 1 millimeter in diameter, Aplysia brain cells are the largest in the animal kingdom, 100 times larger than most human neurons.
In a previous study published in 2011, study coauthor Dr. Yili Zhang had developed a computational model of the biochemical mechanisms behind long-term potentiation, the process by which neurons strengthen their bonds to create long-term memories, in Aplysia. The model was so fine-tuned that it allowed Byrne's team to precisely time when to train sea snail neurons in order to enhance learning.
As a follow-up, they decided to see if the same model could overcome memory deficits, which are often caused by weakened neuron connections.
The first step was to induce memory loss in sea snail neurons. Dr. Rong-Yu Liu, another coauthor, blocked the activity of a gene that produces CREB-binding protein (CBP), which supports long-term memory formation, in the sea snails' sensory neurons. This mimics a rare human condition called Rubinstein-Taybi syndrome, in which a mutation in that gene leads to intellectual and learning disabilities. With CBP production blocked, the snail neurons' connections to each other, and thus their ability to form new memories, were weakened.
The team then used the mathematical model to schedule ideal times for retraining the impaired cells. The idea was that by timing other molecular learning processes, they could strengthen the neuronal connections again and allow the neurons to learn even in the absence of CBP.
Over the course of five precisely timed sessions, at intervals of 5 to 50 minutes, they delivered serotonin, a neurotransmitter chemical that regulates learning.
As the researchers had hoped, the precise timing schedule allowed the neurons to overcome low CBP levels in order to form connections almost as strong as they had been before—once again, the snail neurons could form new memories.
If human brains have biochemical processes similar enough to those of Aplysia sea snails, Byrne believes that his team's findings could eventually lead to a new strategy for strengthening neuron connections and reversing memory deficits in humans with conditions like Alzheimer's disease.
Mathematical models for human brain functions could lead to comprehensive therapies that precisely time cognitive training sessions with the administration of memory-enhancing drugs, in order to maximize the benefits of both.
That's a long way off yet, since much more needs to be understood about the many signaling pathways involved in learning in mammalian neurons.
Still, Byrne's team is optimistic— their paper concludes that "it is likely that progress in many systems will facilitate development of a predictive model describing human [long-term potentiation] and learning."