Scientists Create World's First Atomic X-Ray Laser Beam, Aim to Unravel Molecular Mysteries
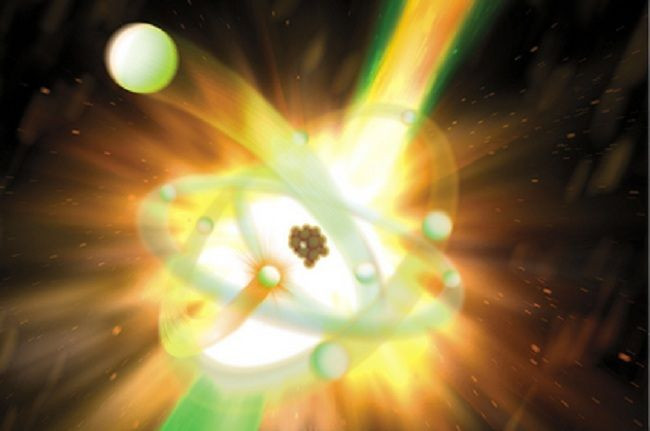
This artist's conception illustrates how the new atomic hard X-ray laser is created. (Illustration: Gregory M. Stewart, SLAC National Accelerator Laboratory)
Government researchers have created the fastest, purest X-ray laser pulses ever achieved, and have fulfilled a 1967 prediction that an atomic scale x-ray laser could be made in the same manner as visible-light lasers., according to a statement released Wednesday.
Researchers at the SLAC National Accelerator Laboratory used the Linac Coherent Light Source to aim a powerful x-ray source beam, a billion times brighter than any previous source, at a capsule of neon gas and triggered an “avalanche” of X-ray emissions to become the world’s first “atomic X-ray laser.”
The new atomic X-ray laser works in the same way as many visible-light lasers and induces electrons to fall from higher to lower energy levels within atoms to release a single color of light in the process.
Researchers said that this achievement was impossible with any other X-ray source but the LCLS, a laser that is capable of energy bursts that exceed the entire output of a small country.
Researchers explained that the LCLS’s powerful X-ray pulses, which are a billion times brighter than any other source, were powerful enough to knock electrons out of the inner shells of many neon atoms contained in the capsule. Following that, when other electrons fell in to fill the holes, about one in 50 atoms responded by releasing a photon in the X-ray range that in turn stimulated neighboring neon atoms to emit more X-rays, creating a cascade effect that magnified the laser light by 200 million times.
Researchers said that the end result is an extremely purified laser beam that will let researchers illuminate and distinguish details of ultrafast particle reactions that have been impossible to see before.
"We envision researchers using this new type of laser for all sorts of interesting things, such as teasing out the details of chemical reactions or watching biological molecules at work," said physicist Nina Rohringer, who led the research. "The shorter the pulses, the faster the changes we can capture. And the purer the light, the sharper the details we can see."
Rohringer said that she will try to create higher energy atomic X-ray lasers with even shorter pulses using oxygen, nitrogen or sulfur gas in future experiments.
LCLS Sizzles Matter to 3.6 Million Degrees, Hotter than the Sun’s Corona
Other researchers working at the U.S. Department of Energy's (DOE) SLAC National Accelerator Laboratory also released a study on Wednesday documenting the power of the X-ray laser.
Researchers from Oxford University fired rapid laser pulses on an aluminum strip 30 times smaller than the width of a single human hair and created “hot dense matter” that was about 3.6 million degrees Fahrenheit, a temperature higher than the sun’s corona, in less than a trillionth of a second.
Scientists added that the aluminum foil heated up so fast it produced conditions of temperature and pressure that are only found in stars.
"The LCLS X-ray laser is a truly remarkable machine," said Sam Vinko, a postdoctoral researcher at Oxford University and the paper's lead author. "Making extremely hot, dense matter is important scientifically if we are ultimately to understand the conditions that exist inside stars and at the center of giant planets within our own solar system and beyond."
Co-author Bob Nagler of SLAC, an LCLS instrument scientist said that the LCLS, with its ultra-short wavelength of X-ray light, is the first that managed to penetrate a dense solid and create an even patch of plasma described as “a cube one-thousandth of a centimeter on a side” - and probe it at the same time.
Researchers said this finding can benefit scientists looking to analyze and recreate the nuclear fusion process that powers the sun.
This photograph shows the interior of a Linac Coherent Light Source SXR experimental chamber, set up for an investigation to create and measure a form of extreme, 2-million-degree matter known as “hot, dense matter.” The central part of the frame contains the holder for the material that will be converted by the powerful LCLS laser into hot, dense matter. To the left is an XUV spectrometer and to the right is a small red laser set up for alignment and positioning. (University of Oxford / Sam Vinko)