Scientists Use CRISPR to Rewrite Defective Muscular Dystrophy Gene In Mice; May Someday Pave Way For Human Treatment
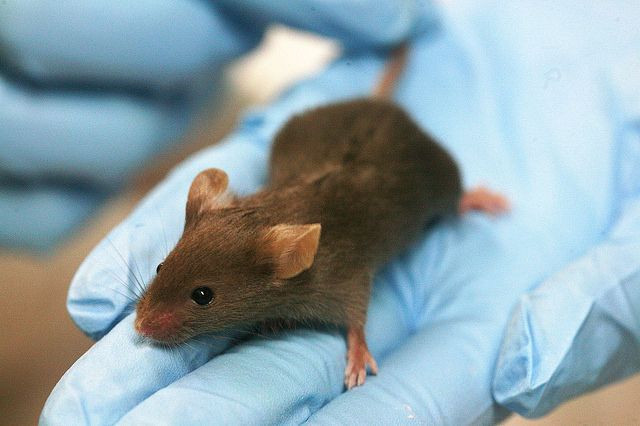
An innovative gene-manipulation technique known as CRISPR-Cas9 has shown further promise as a treatment for Duchenne muscular dystrophy (DMD), all while avoiding potential ethical dilemmas.
A series of three animal studies was published in the journal Science on Thursday, demonstrating the effectiveness of CRISPR-Cas9 in selectively editing the defective gene responsible for DMD, which affects approximately 1 in every 5,600 to 7,700 males ages 5 through 24 in the U.S. The researchers used a harmless virus (adeno-associated virus, or AAV) to inject the CRISPR-Cas9 gene editing system into living mice; once inside, it was able to snip away one of the genetic mutations thought to be responsible for DMD. Afterwards, these injected mice had a degree of their muscle function restored, in some cases for as long as six months.
“We are very encouraged by these results in animals and feel this work is an important step forward in realizing the potential of gene editing to correct disease-causing mutations in muscle tissue of patients with DMD,” Amy Wagers, one of the many study authors and a Harvard University researcher, told Medical Daily.
Muscular dystrophy describes any number of conditions that result in the progressive degradation of muscle throughout the entire body; DMD is the most severe and well-known. Those afflicted with DMD—almost always boys since the gene responsible is found on the X chromosome, and girls can be perfectly healthy living with one mutated version and one normal copy—only have an average life expectancy of 25 and will invariably lose the ability to walk long before then.
In DMD, production of the protein dystrophin, which ensures muscle stability, is stopped entirely. Dystrophin, while essential, isn’t a zero-sum affair, though—if a treatment could jump-start a DMD sufferer’s production to even 20 to 30 percent of the level found in healthy individuals, it’s believed they could live a symptomless life. Even a 4 percent boost, similar to what’s seen in people who suffer from less severe forms of muscular dystrophy, could possibly add better-lived decades onto their lifespan, along with less heart damage.
CRISPR is an acronym for “clustered regularly interspaced short palindromic repeats.” Found in many microorganisms, it’s a segment of DNA that repeats itself while containing unique DNA bits nestled within its open spaces. These bits are actually the DNA of past viral foes and act as a rudimentary immune system. When the microorganism comes across that virus again, its Cas (CRISPR-associated protein) springs into action and very precisely attacks the hapless invader, slicing its DNA into ribbons. Scientists discovered they could rejig the CRISPR/Cas system to target most any piece of DNA, allowing them to precisely edit the genes of a cell.
The authors of each study used a modified CRISPR-Cas9 system, one with a sleeker Cas9 protein able to fit itself inside the AAV. The virus, geared specifically to infect muscle cells, would then deliver the system to the cell’s dystrophin gene located within. Its target? One of the many regions of the gene containing instructions to make the protein known as exon 23. When exon 23 is mutated, it prematurely terminates dystrophin production; the researchers hoped that by deleting it, they would give the gene the ability to repair itself and create a “truncated” but functional version of dystrophin.
The first study, led by Christopher Nelson of Duke University, found that injecting their CRISPR-editing software into the lower leg of their mice subjects led to the deletion of exon 23 from 2 percent of affected muscle cells; that in turn restored dystrophin levels to about 8 percent of normal production. Further research found that this restoration lasted for about six months.
The study conducted by Wagers and her team, which used fluorescent markers to better track their gene editing process, found similar improvements in muscle cells directly infected by the AAV and, more crucially, evidence of exon 23 deletion in the satellite cells nearby as well. These satellite cells act as muscle stem cells, according to Wagers.
“[I]f gene editing did not occur in satellite cells, then every time the muscle was damaged and satellite cells were activated to contribute to muscle repair, the new nuclei added to fibers would still contain the original DMD mutation, which does not produce a functional dystrophin protein,” she explained. Their findings indicate the gene therapy can “create a pool of regenerative cells” that continue to provide dystrophin for long stretches of time, a decisive advantage over other potential gene treatments that attempt to repair a person’s specific DMD mutation but would likely need to be given very regularly.
“The benefit of gene editing over other gene therapy techniques is that it can permanently correct the 'defect' in a gene rather than just transiently adding a 'functional' one,” Chengzu Long, lead author of the third study and researcher at the University of Texas Southwestern, told Medical Daily.
Long and his colleagues experimented with a variety of different methods to deliver their CRISPR-AAV package. Using newly born mice, they injected their gene-editor into the abdomen, the back of the eye, and muscle tissue. While each method had varying levels of success, dystrophin expression in vascular smooth muscle cells was best seen in mice who had received the therapy through their eyes. Though muscle tissue injections are useful for proving effectiveness, the holy grail of DMD gene therapy will necessitate restoring dystrophin throughout the body.
“Because DMD affects almost all muscle groups, and the heart, a systemic therapy is much more attractive,” said Wagers. All three teams were able to trigger some degree of systemic restoration in their mice.
A previous experiment led by Long demonstrated that a similar technique, performed on mouse embryos, would eliminate exon 23 from 80 percent of newly born mice. But what makes the findings published Thursday—the first to be seen in live animals—especially promising is that they avoid directly tampering with reproductive cells.
Though gene-editing embryos with a DMD mutation has been floated in recent years as a way to effectively prevent the disease, there are many in the research community, and elsewhere, who feel it would cross ethical boundaries, potentially opening up the door to all sorts of questions about genetically modifying human embryos. The current method, on the other hand, could someday allow for the treatment of those who have already come down with DMD and other genetic disorders.
“We were pleasantly surprised that the CRISPR gene editing system worked as intended with relatively little optimization, and I am optimistic that continued development of these very early experiments will make the approach even better,” Charles Gersbach, one of Nelson's co-authors and a Duke University researcher told Medical Daily. “It is also reassuring that all three groups reached the same conclusion with similar, but slightly different, approaches. This is a testament to the robustness of the technology.”
Encouraging as these findings are, there are many more hurdles to climb. For example, none of the delivery methods, whether local or systemic, can currently pass through the blood-brain barrier, preventing dystrophin restoration in the brain.
“There needs to be continued optimization, as well as scale up to larger animal models. But most importantly safety needs to be demonstrated and responses from the immune system need to be better understood,” Gersbach explained. “This will likely take years, but if successful then clinical trials could be envisioned in the foreseeable future.”
That future does certainly appear brighter than ever before, though. Long’s research group at UTSW, for instance, recently received a five-year, $7.8 million funding grant from the National Institutes of Health to open up a research center dedicated to translating “scientific findings and technological developments into novel treatments for muscular dystrophy.”