Tooth Enamel Doesn’t Regenerate But Lasts A Lifetime — Here’s Why
Tooth enamel is the hardest substance in the human body. It has to be, given the relentless demands made on our teeth daily to sustain life by chewing, grinding and tearing up food. And why can tooth enamel outlive us?
That's the question an American research team sought to answer -- and did -- thanks to some pretty brainy thinking and some really cool technologies. Basically, the reason why our tooth enamel is stronger than our bones is because the imperfections in tooth enamel taken together create a crystal substance that ranks fifth on the Mohs scale of mineral hardness.
This scale measures the scratch resistance of various minerals through the ability of harder material to scratch softer material. A rank of one is the softest (talc) and number 10, the hardest (diamond). In this scale, our tooth enamel is ranked as an "apatite,” which is as tough as obsidian or volcanic glass. Apatite is the appropriate word for tooth enamel, by the way.
More specifically, the authors of a study about tooth enamel published recently in the journal, Nature Communications, found that enamel's secret lies in the imperfect alignment of the many "hydroxyapatite crystals" that are the building blocks of a tooth's enamel rods. These enamel rods are long and thin, and are around 50 nanometers wide and 10 micrometers long.
Led by Prof. Pupa Gilbert from the University of Wisconsin-Madison, the team also consisted of members from the Massachusetts Institute of Technology (MIT) in Cambridge and the University of Pittsburgh.
Prof. Gilbert took a detailed look at the structure of enamel to determine how tooth enamel manages to stay functional and intact for more than a lifetime.
"How does it prevent catastrophic failure?" was one of the key questions the research team sought to answer, said Prof. Gilbert.
The use of cutting edge imaging technology in the research was a given but wouldn't answer the question completely. For that, the research team had to rely on a technique called "polarization-dependent imaging contrast (PIC) mapping," which Prof. Gilbert designed, to visualize how individual crystals in tooth enamel are aligned.
The research team acknowledged that before the advent of PIC mapping, it would have been impossible to study enamel with this level of detail.
"You can measure and visualize, in color, the orientation of individual nanocrystals and see many millions of them at once," Prof. Gilbert explained about the edge given the team by PIC mapping.
"The architecture of complex biominerals, such as enamel, becomes immediately visible to the naked eye in a PIC map."
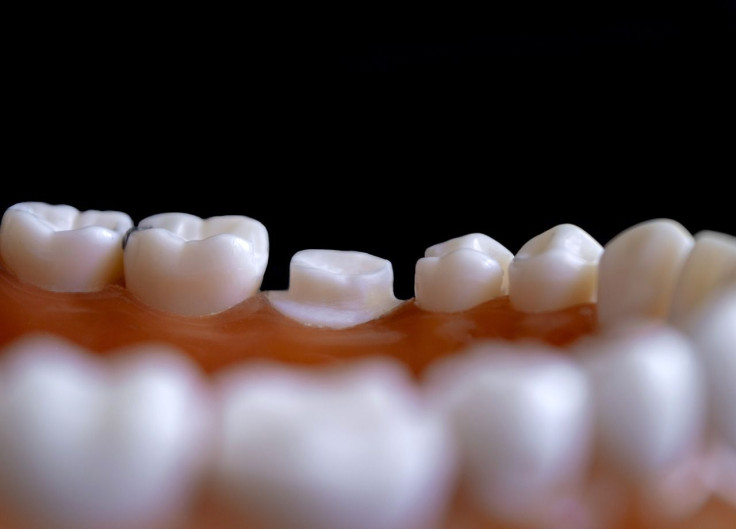
Researchers uncovered patterns when viewing the structure of enamel using PIC mapping. "By and large, we saw that there was not a single orientation in each rod, but a gradual change in crystal orientations between adjacent nanocrystals," according to Prof. Gilbert. "And then the question was, 'Is this a useful observation?'"
To test if the change in crystal alignment influences the way that enamel responds to stress, the team used a computer model simulating the forces hydroxyapatite crystals experience when a person chews.
Researchers placed two blocks of crystals next to each other so the blocks touched along one edge. Crystals within each of the two blocks were aligned, but the crystals met at an angle where they came in contact with the other block.
In several trials, researchers altered the angle at which the two blocks of crystals met. They found a crack was formed when they applied pressure if they perfectly aligned the two blocks at the interface where they met.
When the crystals were only slightly misaligned, however, the interface deflected the crack and prevented it from spreading.
"If there is an ideal angle of misorientation, I bet it's the one in our mouths," she mused.
Study co-author Cayla Stifler sought to prove this by returning to the original PIC mapping information and measuring the angles between adjacent crystals. After incredibly painstaking work, Stifler found that one degree was the most common size of misorientation. The maximum misorientation was 30 degrees.
This observation agreed with the simulation. The conclusion: smaller angles seem better able to deflect cracks.
"Now we know that cracks are deflected at the nanoscale and, thus, can't propagate very far. That's the reason our teeth can last a lifetime without being replaced," Prof. Gilbert concluded.